Removing the brain from the skull in brain banking: much more than you wanted to know
It’s time to think outside of the box
To study the brain, especially areas that cannot be easily reached by biopsies, we need to remove it from the skull. Extracting the brain specimen is also very helpful for shipping it and minimizing storage costs. But what’s the best way to extract the brain?
It turns out that — like seemingly everything in the field of brain banking — there are lots of different ways to remove the brain from the skull. They each make more or less sense in different circumstances.
Tools of the trade
But first, let’s talk gear. All methods of removing the brain intact rely on cutting through the skull. Historically, the tools used for cutting into the skull tended to be blunt instruments:
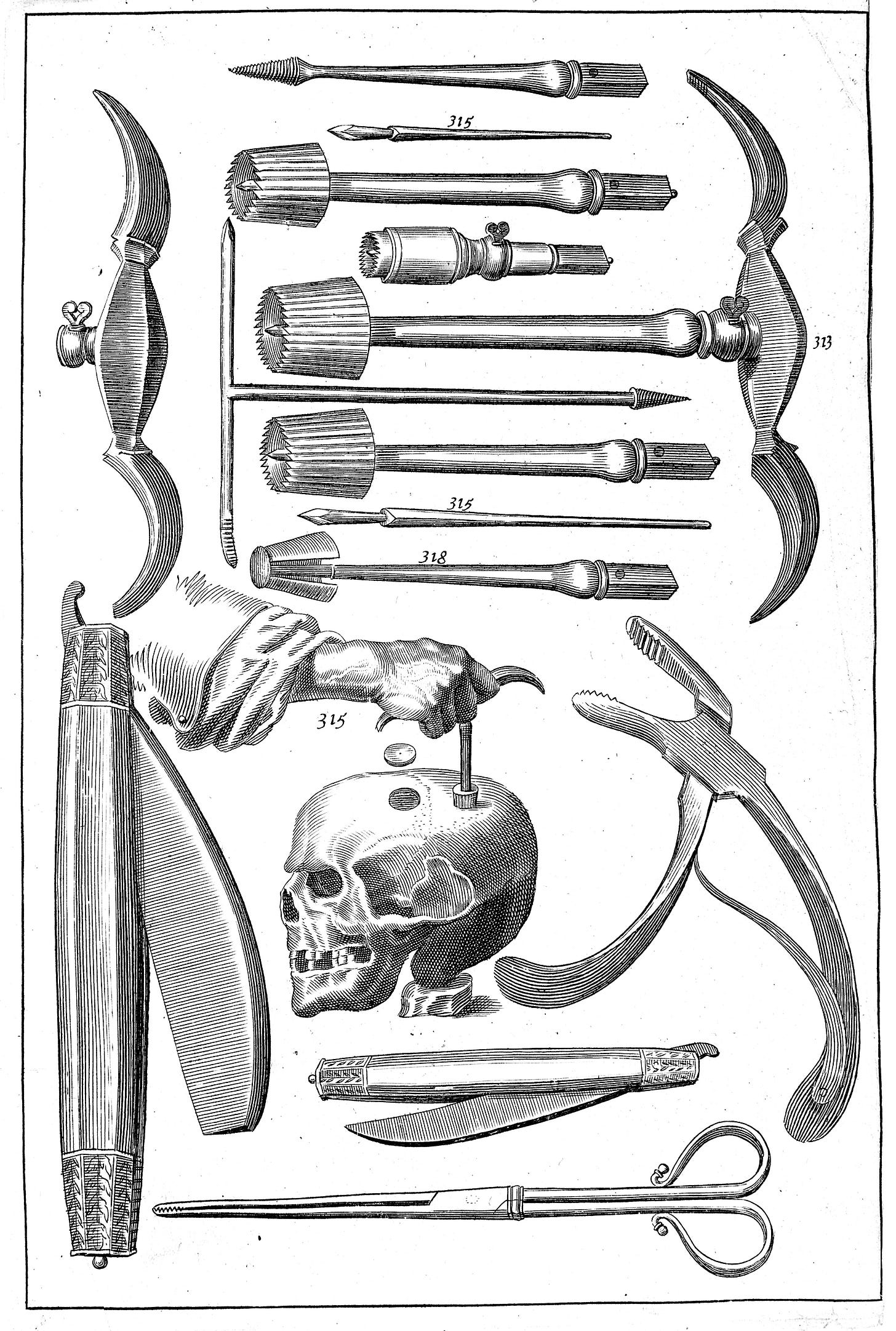
These would still work today, but it would take considerably longer to cut the skull this way. Instead, power tools are generally preferred.
In modern neurosurgery, precise cranial drills are often used:
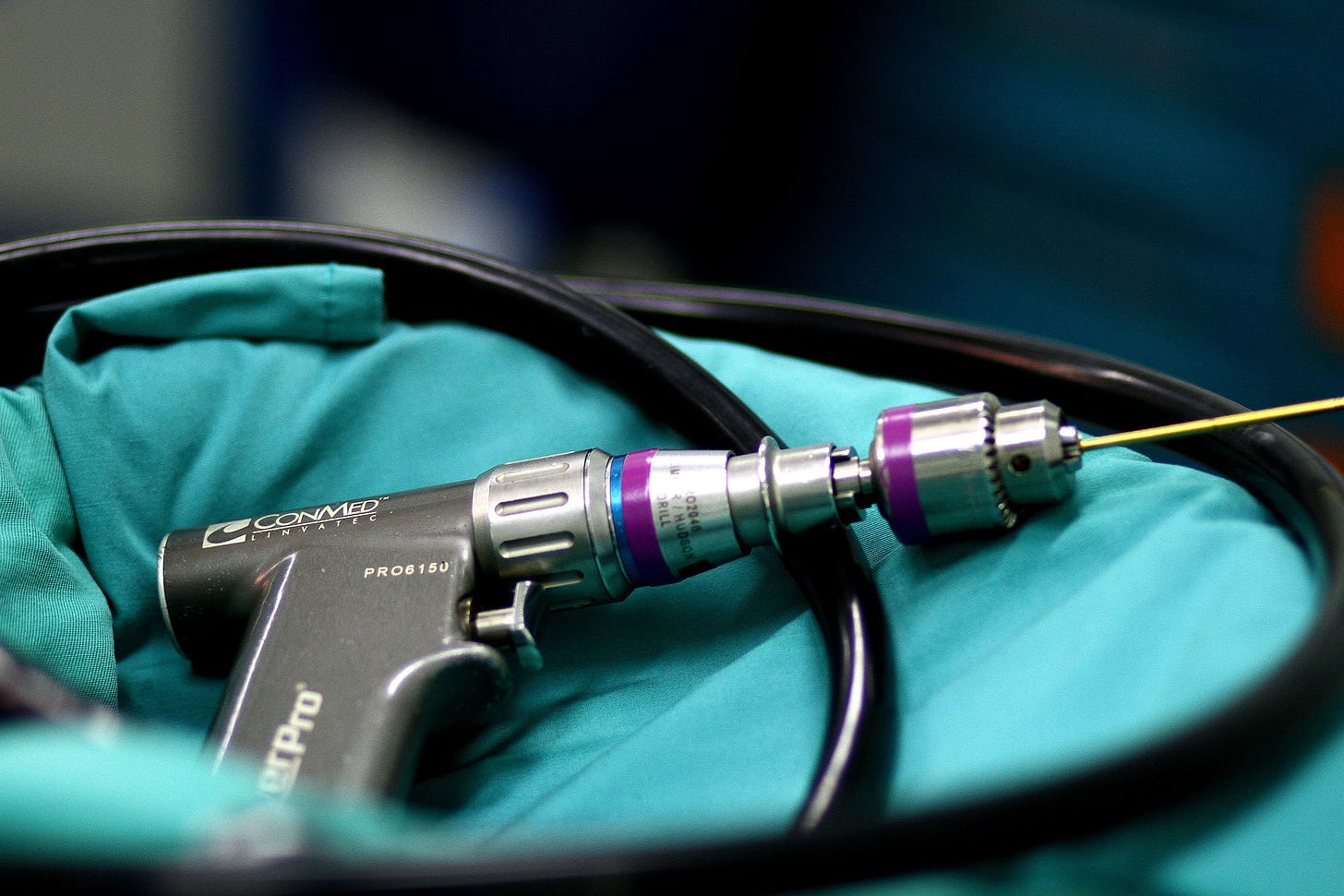
Cranial drills cut into the skull relatively slowly, which is a feature insofar as they’re less likely to accidentally cut into brain tissue, but a bug insofar as they take a longer time to access the brain.
This trade-off makes sense in most neurosurgical applications because the brain is (usually!) still being perfused, but it doesn’t make as much sense in brain banking. Once the person is dead, the delivery of oxygenated blood has stopped and the brain is beginning to decompose, making speed of the essence.
(Also, I should note, brain banking procedures don’t reimburse at a rate of $100,000 like some neurosurgical ones do. But this is a side point. The main reason to go faster in brain banking is that the brain is decomposing, unlike almost all neurosurgical procedures.)
So, because of the need for speed, brain bankers generally use bone saws. These are also called autopsy saws. For example, Long 2014 use the Mopec Swordfish Autopsy Saw (which, as of today, costs $3000):
One of the issues with using a bone saw is that it can be hard to prevent going through the skull into brain tissue at the end of the cut. Some bone saws have features meant to minimize this risk. Additionally, some brain bankers use a hammer and chisel to finish the cuts and remove the skull once it is mostly cut. Hlavac 2018 touch on this in describing their use of a bone saw:
[A]n advanced ultrasonic surgical bone saw (SONOPET, Stryker Model #UST-2001) was used for a portion of the laminectomy and craniotomy in the first dissection. This saw is designed for fine bone dissection during live surgical procedures and has an auto-stop feature upon contact with soft tissue. However, because of its fine precision, it proved to be a less efficient approach for the extent of cutting required for a full laminectomy. Thus, the traditional bone saw [AM: Shandon Model #10000], followed by hammer and chisel, was determined to be both sufficient and efficient. Moreover, the haptic feedback from the hammer and chisel was helpful to avoid damage to the nervous tissue.
There’s more than one way to extract a brain
Cutting through the skull is not the easiest thing in the world, but it’s not the hard problem of brain extraction. The hard problem is dissecting away the tethers connecting the brain to the rest of the body — the cranial nerves, the blood vessels, the pituitary stalk, and the spinal cord — so that the brain can be carefully removed without causing tears.
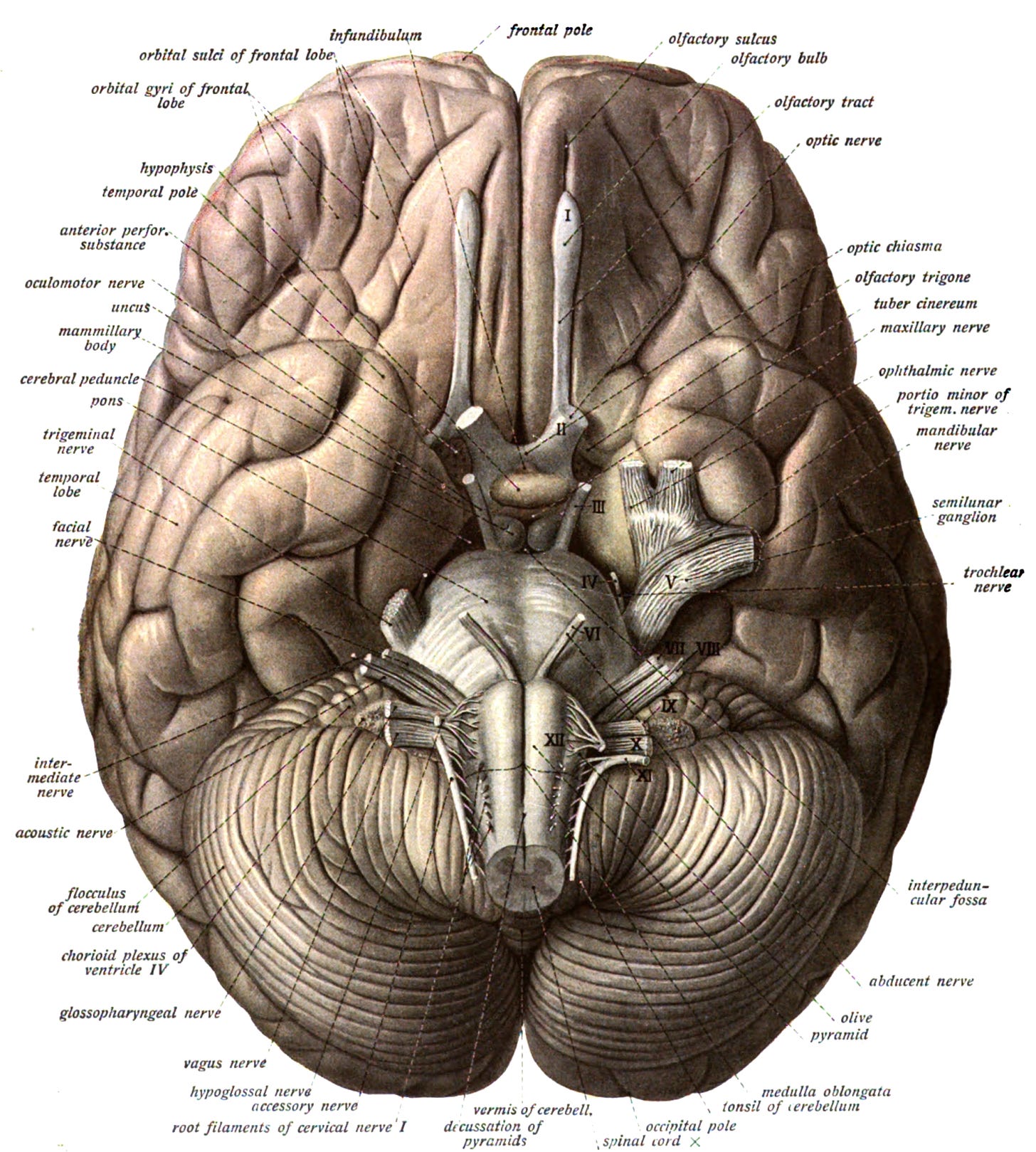
There seem to be two main approaches to this problem: one is an approach going from the front of the brain only, which I’m calling the anterior-only approach. The other is an approach going first from the back of the brain and then from the front of the brain, which I’m calling the combined posterior-anterior approach, or the “combined approach” for short.
Anterior-only approach
This is the most common method used for brain extraction, which is described in various standard anatomy textbooks.
Basically, the skullcap (also called the calvaria) is cut and removed. The brain is then slowly lifted up from the anterior side — specifically, the frontal lobes are elevated — while the cranial nerves, arteries, and pituitary stalk are cut. As these tethers are cut, the brain continues to be lifted up until all of its connections, including its connection with the spinal cord, are finally cut and it can be removed from the skull.
Here’s a much more detailed description from an anatomy textbook if you’re interested:
In this case, a video is worth more than a thousand words, and there are some YouTube videos showing this dissection process. It’s important to note that these videos are age-restricted and will be graphic for people who are not used to viewing autopsies. You can find examples of these videos here, here, and here.
Combined posterior-anterior approach
In addition to the standard method, there are a few iconoclast articles pointing out that the anterior-only approach has limitations and suggesting a posterior-anterior approach instead. Examples of this are Felle 1995, Long 2014, and Hlavac 2018.
A core difference of the combined approach is that a cut is also made into the occipital part of the skull:
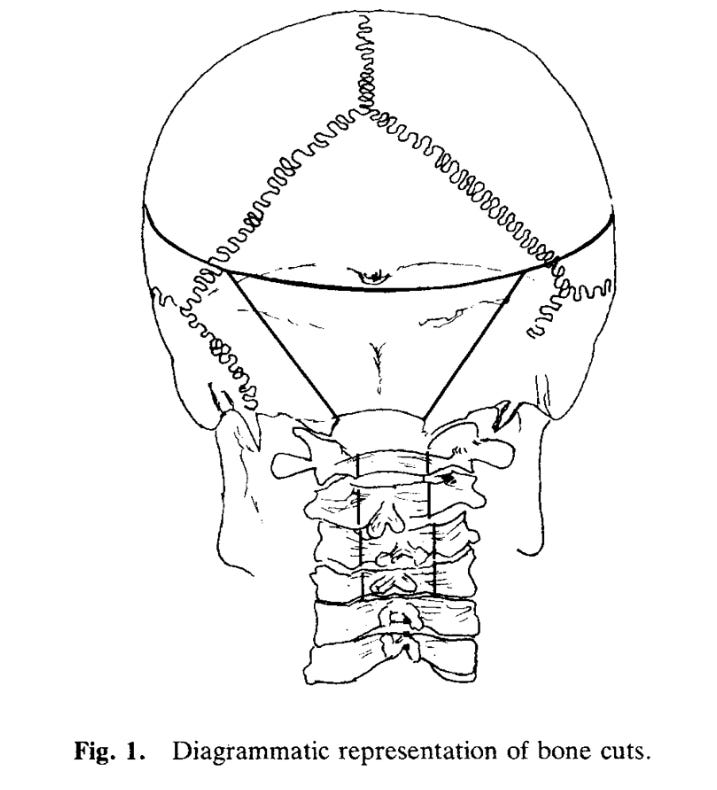
By allowing access to the back of the brain, the posterior tethers of the brain, such as the tentorium cerebelli and cranial nerves V-XII, are able to be cut from a posterior viewpoint. These dissections can be more precise because it’s easier to see what is being done.
Once the posterior tethers have been cut, the brain is turned around and the anterior tethers are cut after elevating the frontal lobes.
Because the spinal cord is on the posterior side of the body, this also means that more of the spinal cord can be preserved, rather than haphazardly sectioning it from the brain in the anterior approach. Or, in the method of Hlavac 2018, allowing for the whole spinal cord to be removed intact along with the brain. Of course, retaining the spinal cord also requires the removal of the laminae of the vertebral column, to allow access to the spinal cord from the posterior aspect:
Felle 1995 describe some of the trade-offs of the combined approach compared to the standard anterior-only approach:
The method described is somewhat more difficult than the traditional one (Romanes, 1986). It requires, in addition, resection of a wedge of squamous occipital bone and the laminae of the upper cervical vertebrae. However, subsequent dissection of the cranial nerves, pituitary stalk and internal carotid arteries is rendered easier as these structures are better visualized. Moreover, specimens include the complete brainstem and upper cervical spinal cord, the lower cranial nerves, and the spinal root of the accessory nerve.
Long 2014 describe a key upside of this approach:
Keeping the bulk of the brain in situ for the majority of the procedure means that the brain does not have to be reflected to the same degree as required by the traditional approach; also, its weight is supported by the three cranial fossae.
One downside of this approach, at least the way Hlavac 2018 perform it, is that it requires flipping the cadaver over several times. Anyone who has viewed or participated in surgical procedures knows that this is one of the most annoying and confounding parts of any surgery.
Table comparing the anterior-only approach with the combined anterior-posterior approach
The words “more” and “less” in describing these trade-offs are weasely for a reason — the degree to which they will occur is not only uncertain but also depend on the context. Here is a couple of those relevant variables.
Extracting the brain while fresh or fixed
One of the most relevant variables to this discussion is whether the brain is fresh or fixed. “Fresh” in this context means not fixed. Fixation is usually with formaldehyde or another aldehyde-based fixative. Fixation can be performed via perfusion or by removing the skullcap and dura and then using diffusion- or convection-based techniques to fix the brain in place.
If the brain is fresh, then the traumatic damage from manipulating it will cause more microscopic damage.
What are the microscopic sequelae of traumatic removal of the brain? It can cause:
1: Compacted neurons. These are often called "dark" neurons because they stain dark color in response to certain dyes, including being hyperbasophilic on H&E, because their constituent biomolecules develop excess negative charges.
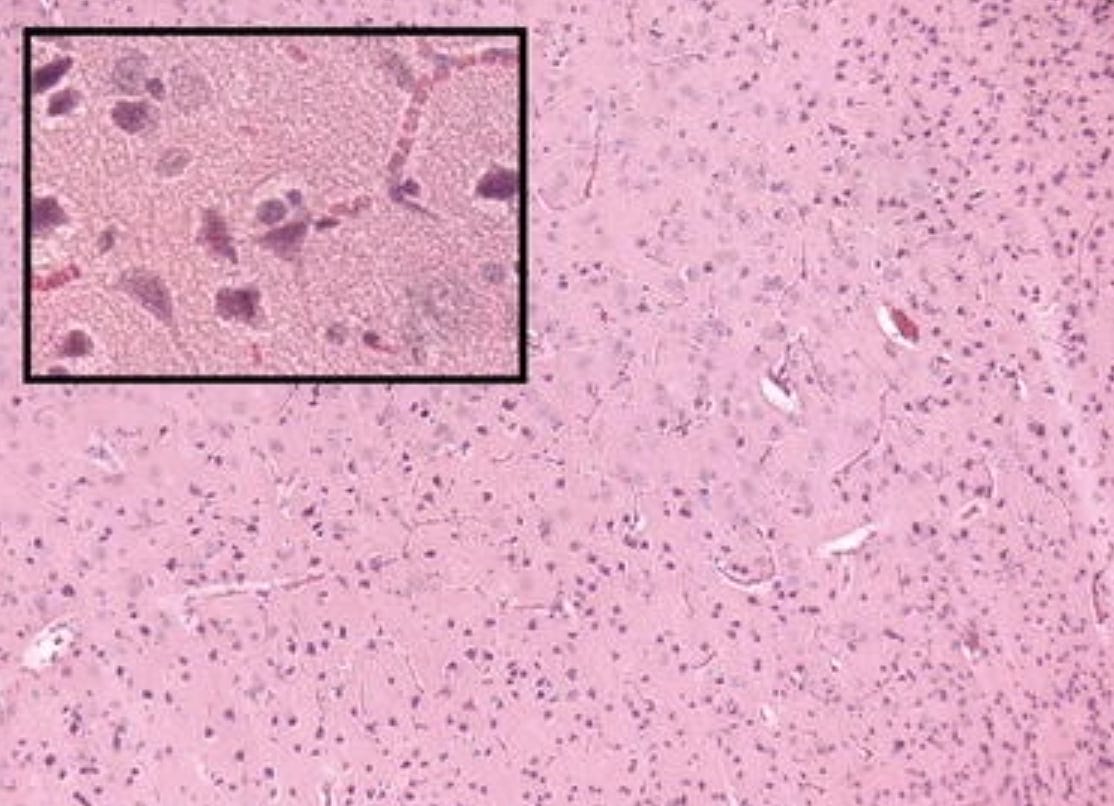
Much more on compacted neurons in a footnote, which most people will likely not be as obsessed with as I am1. To summarize, compacted neurons are probably the most common microscopic artifact that will occur when fresh or insufficiently fixed brain tissue is removed from the skull, because of a gel-gel phase transition caused by the trauma. They occur haphazardly, certainly not in all neurons. They are absolutely to be avoided if possible and would affect some investigations, like cell volume measurements. But this artifact has been found to be reversible even in fixed tissue and it does not seem that it would prevent tracing of connectivity or significant information loss when using advanced imaging and reconstruction techniques.
2: Buscaino bodies. These are associated with traumatic handling of the brain postmortem and are thought to be caused by the solubilization of some component of myelin. Also problematic, but also do not seem too damaging from an information perspective.
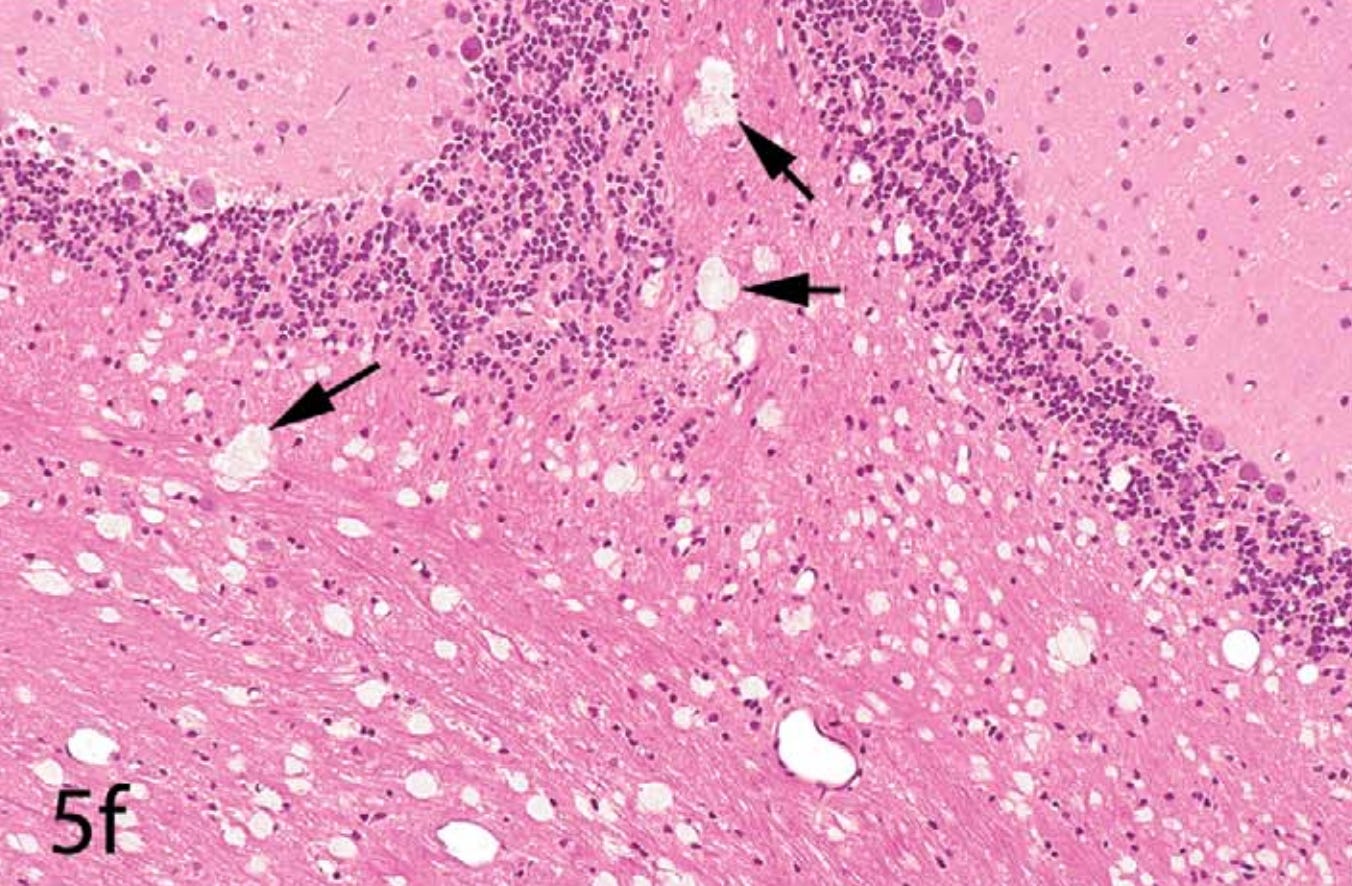
3: Separation of layers and other mesoscale artifacts. This can occur as a result of forcible removal of the skull and might require computational techniques to reverse such as registering and stitching the layers back together.
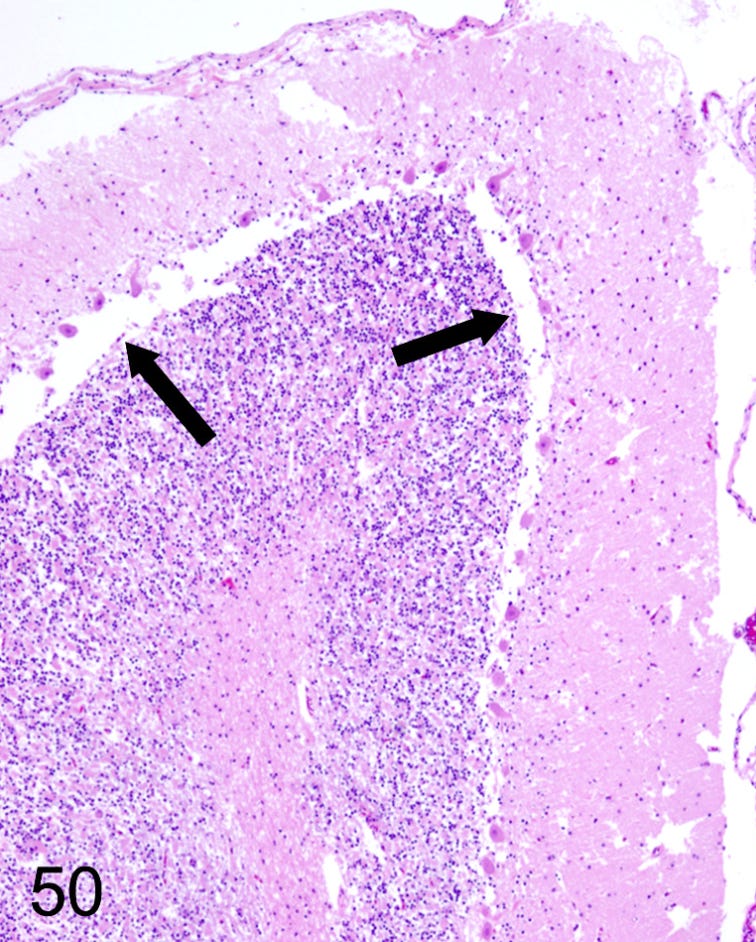
4: Probably other artifacts — I haven’t seen a comprehensive study or review on this topic.
5: It’s worth pointing out that the majority of human brains are studied after the removal of the fresh brain from the skull. While there are known artifacts resulting from this procedure, especially compacted neurons, it seems that the microscopic brain structure is sufficiently well maintained even after the removal of the fresh brain from the skull, at least in most cases.
It’s also worth pointing out a downside of fixing the brain prior to removal. If the brain is fixed, then it will be more difficult to move or reflect the brain as is needed to dissect away its tethers and extract it from the skull. This might necessitate more aggressive maneuvers and lead to more macroscopic damage to the brain.
Using the combined approach makes relatively more sense in fixed brain tissue
There are a couple of trade-offs of using the combined approach that make it particularly compelling if the brain is fixed:
The combined approach takes longer. In fresh tissue, this is a big problem because the brain will be decomposing throughout — as the adage goes, time is tissue. On the other hand, if the brain is fixed, decomposition will be dramatically slowed or stopped, so the extra time required is much less of a problem.
The combined approach doesn’t require the brain to be moved or reflected as much to be able to dissect away the brain’s tethers. Because moving the brain is more difficult in fixed tissue, which is stiffer, removing the fixed brain will be relatively easier using the combined approach than with the anterior-only approach.
A couple of studies have already reported that they removed the brain with the combined approach following fixation: Hwang 2006 and Long 2014 both perfused fixatives prior to using the combined approach.
One note is that most contemporary brain banks usually want to remove the brain while fresh, in large part because they want to cryopreserve at least part of it while fresh for molecular biology studies that require non-fixed tissue. This means that the combined approach is less compelling, which may be part of why it isn’t as commonly used.
Degree of brain decomposition prior to the start of the procedure
Another important variable is the degree of decomposition that has occurred in the brain before the procedure begins. More decomposition makes the brain softer and thereby harder to remove intact while fresh.
Within the field of forensic neuropathology, there are ways to qualitatively score the degree of decomposition based on the gross morphology of the brain.
In one such scoring system, one of the criteria is that as the decomposition score increases, removal of the brain becomes more difficult. At the lowest decomposition score, the brain is able to be extracted without fragmentation. As the brain decomposition score increases, the surface and then the internal parts of the brain soften and eventually turn into to a liquid. This means that the brain is more difficult to remove fresh without fragmentation. At the higher decomposition scores, the brain disintegrates or flows out when the dura is excised, entirely preventing it from being removed intact.
Most of the brains with severe decomposition scores are not good candidate specimens for brain banking anyway. And ideally, more decomposed brains would be fixed in situ prior to removal, to help make the brain stiffer prior to removal.
If the brain is going to be removed fresh despite substantial decomposition, it’s unclear which method would be better. But it’s plausible that the anterior-only approach might be better because the brain will be preserved more quickly with less overall handling time.
Putting it all together
As I discussed in a previous post, I like to think of brain banking procedures as falling into two main contexts: the kinds of procedures that can be performed in a well-resourced central location, and the kinds of procedures that can be performed in a scalable way in funeral homes prior to shipping the brain to the brain bank.
For a well-resourced brain bank, the ideal procedure would probably involve in situ fixation and the use of the combined approach for brain removal. This minimizes microscopic damage during the removal process, minimizes decomposition time, and allows a large amount of tissue to be preserved intact, including longer cranial nerve stubs, whatever amount of spinal cord is desired, and possibly eye tissue as well.
Fixation isn’t binary, though — it’s always a matter of fixation degree. As the tissue is exposed to aldehyde and fixation continues over time, brains are likely to become more difficult to maneuver. There is an open question of how much fixation the brain can undergo before removing it even with the combined approach — which still involves maneuvering and reflecting the brain — is excessively challenging and leads to macroscopic damage.
For scalable procedures performed in a funeral home, a key problem is finding someone who can remove the brain from the skull for the purpose of preserving it at all. It’s certainly not something that most people would feel comfortable doing.
In other words, in a lot of circumstances, this whole discussion is a bit of a moot point, because the autopsy technician available locally may only know how to perform the brain extraction procedure with the standard anterior-only approach.
The Brain Support Network helps with identifying cooperative funeral homes and pathology specialists who can perform brain removal and fixation in many locations around the US. Their FAQ notes that — in most states — performing a brain procurement procedure does not require a license. They report that New York is an exception, where an MD license is required.
Even if the standard anterior-only approach is the only procedure that can be performed in this setting, it’s still helpful to be aware of how the procedure works and what some of its limitations might be.
Technological improvements could also help with brain extraction procedures performed in funeral homes, such as the development of better but still practical tools and training procedures.
Compacted neurons have been called the most common artifact found in fixed brain tissue, so it’s worth spending some time considering how damaging they are likely to be to neural information.
They have a shrunken cell body, a shrunken nucleus, and they have slender, shrunken, and "cork-screw"-like dendrites. The transition from normal to compacted neuron happens throughout the soma-dendrite compartment as an all-or-nothing phenomenon. It also happens in the axon.
Compacted neurons shrink to a significant degree. The mechanism seems to involve rapid loss of water (perhaps up to 50% of cellular water) and a gel-gel phase transition.
They primarily occur as a result of mechanical trauma, for example occurring when removing the brain from the skull fresh prior to fixation or in adjacent regions found near cuts of the fresh brains.
Their distribution can appear haphazard — the compaction phenomenon certainly does not occur in all neurons.
In vivo, the formation of compacted neurons appears to be reversible. Indeed, some data suggest that reversibility is the expected outcome, assuming that the underlying cause has been resolved. In one study in rats, the "overwhelming majority" of neurons that were compacted by external forces recovered normal morphology within 4 hours. Compacted neurons can also be reversed with proteinase K enzymatic treatment of fixed tissue sections.
Under the electron microscope, compacted neurons don't seem to involve damage to cell membranes. Instead, they seem to be due to a "dramatic compaction of apparently undamaged ultrastructural elements" without inducing apparent discontinuities of the plasma membrane. Synapses are still present.